Hazardous materials incidents can expose emergency responders to dangers that require the use of air-monitoring instruments to operate safely. These dangers include thermal, radiation and oxygen concentrations and chemical hazards.
Thermal hazards involve materials that are flammable and those that are very cold. Cold materials are usually cryogenic liquids that are generally not toxic, but have large liquid-to-gas expansion rations, so small leaks can produce large vapor clouds. These vapor clouds can displace oxygen in the air and cause asphyxiation. Monitoring with oxygen meters can determine whether there is enough oxygen to breathe safely.
Flammable materials of most concern are those that are liquids or already gases. Liquid flammable materials produce various levels of vapor, depending on the physical characteristics of the material. Most flammable vapors are not visible to the naked eye and monitoring is necessary to determine whether the materials are present, where they are located and if they are in the flammable range. Chemical hazards include toxic, corrosive and reactive materials.
Using monitors at hazmat scenes
Most serious injuries and deaths involving airborne releases occur through exposure to toxic materials. Toxic materials can enter the body through ingestion, skin contact, injection and inhalation. The primary reason for wearing chemical protective equipment on the scene of a hazardous materials incident is to provide protection against toxic materials. In order to select proper personal protective equipment (PPE) and operate safely around hazardous materials at an incident scene, responders use air-monitoring instruments to determine the type of chemical present and where it is located.
The Reno, NV, Fire Department’s Hazmat Operational Procedures emphasize analyzing and monitoring incident scenes to conclude incidents more quickly and shrink the required distances of “hot zones.” Using this process, responders have reduced the average incident on-scene time from six hours to 45 minutes.
Reno hazmat team members are trained to be analysis “experts.” A primary tool for on-scene analysis is a laboratory-grade, portable gas spectrometer that identifies liquids and gases. This is an expensive instrument and few fire departments can afford them. Reno hazmat technicians operate a shop for testing, calibrating and maintaining the department’s monitors.
Robots are increasingly becoming a part of our society from manufacturing to helping with chores at home. Bomb squads have used robots extensively for years to handle dangerous jobs, no doubt saving the lives of countless bomb squad members. Robots can also be used at hazmat incidents for recon and air monitoring to keep personnel out of harm’s way. Fire departments across the country use specially designed robots that are dedicated to hazmat response or used for both hazmat and bomb squad operations. For example, Picatinny Arsenal Fire and Emergency Services in New Jersey uses a robot with many features tailored specifically for hazmat responses. These features include a chemical/biological meter, multi-gas meter, radiation detector, laser temperature detector and a manipulative arm with a griping claw. Readings received from the meters on the robot are logged into a computer-type device.
Selecting meters
To select meters, determine potential hazards in your jurisdiction from transportation exposures and fixed facilities. Hazards can include oxygen-deficient atmospheres such as confined spaces, flammable liquids and gases, radiation and toxic materials. Identify and select monitoring instruments that are easy to calibrate and maintain. Establish standard operating procedures (SOPs) for using monitors and identify action levels.
Action levels are concentrations of a gas or vapor in air that require a certain response from personnel operating in the atmosphere. For example, the U.S. Occupational Safety and Health Administration (OSHA) sets an action level of 10% of the lower explosive limit (LEL) when operating in a potentially flammable atmosphere. When 10% is reached, which is considered a potentially explosive atmosphere, action must be initiated to protect personnel operating in that atmosphere. This may include ventilation and PPE. If 25% of the LEL is reached, withdraw personnel and take precautions for dealing with an explosive atmosphere.
Upon arrival on the scene of a potential hazmat incident, one of the first actions to take for a known or unknown material is air monitoring. Personnel using monitoring instruments must be trained in their use. Instruments must be calibrated according to manufacturers’ instructions before use and in some cases following use before being stored. If unidentified materials are encountered, first monitor the air for corrosive gases with pH paper to ensure instrument operation will not be affected by the gases. Next, monitor the air for oxygen concentration, radiation and flammable and toxic atmospheres. Instruments are available that can monitor for oxygen and LEL as well as other gases at the same time. Combustible-gas monitors require sufficient oxygen to operate properly to determine LEL. All monitoring instruments have operating condition limitations and personnel operating them should be aware of those limitations.
Technologies of monitoring instruments
Combustible gas indicators (CGI) measure the concentration of a combustible gas or vapor, using catalytic bead technology, commonly a Wheatstone Bridge. Detection is accomplished by a platinum filament that is heated by burning the combustible gas or vapor. The increase in heat is measured and a readout displayed that is a percentage of the material’s LEL. Effective use of this and all monitoring instruments requires that the operator understand the operating principles and procedures. Maintenance involves recharging or replacing batteries and calibrate immediately before use. The monitor can be used as long as the battery lasts or the recommended interval between calibrations, whichever is less. Limitations include lack of valid readings under oxygen-deficient conditions and filament damage by certain compounds such as silicones, halides, tetraethyl lead and oxygen-enriched atmospheres.
Electrochemical indicators monitor toxic-gas and oxygen-deficient atmospheres. These are single-sensor monitors for each of the materials being measured. These sensors use an electrolyte solution, which is a chemical that, when dissolved in water, conducts electricity. The solution is stored inside the sensor behind a permeable barrier. Two electrodes in the solution sense electricity.
Electrochemical sensors are designed for a specific chemical such as chlorine, ammonia, carbon monoxide, oxygen, nitrogen and sulfur dioxide. When the chemical diffuses across the barrier, it dissolves in the solution and contacts the sensing electrode, which produces ions and electrons. These travel to the counting electrode and are counted, resulting in a percentage reading in the case of oxygen, direct digital readout or an alarm based on a preselected value is reached or exceeded. Limitations include: similar chemicals may cause false positives; gases detected may poison the sensor’s electrolyte solution; particles or condensation from hot gases or water vapor can clog the sensor membrane; and going from low to high humidity may inhibit the movement of molecules across the membrane.
MOS technology
Metal oxide sensor (MOS) technology involves the use of a metal oxide and semiconductors to sense a particular gas. Contaminants change the conductivity of the semiconductor. They can sense multiple substances at the same time. Limitations include non-selective sensors and only provide accurate quantitative data for the calibration gas. These monitors are product specific, giving a ppm readout and provide a percent of the LEL. They are used for broad-spectrum general sensing.
Photoionization detectors (PIDs) are used to monitor organic and some inorganic gases and vapors. The sensor technology involves a sample drawn into a chamber. It is then exposed to ultraviolet (UV) light and the light ionizes the contaminant. Ions allow an electrical current to flow and the greater the number of ions, the stronger the current. The monitor interprets the ion flow as the parts per million (ppm) of the chemical. Limitations of the detector include: it does not monitor for methane; response may change when gases are mixed; dust; needs proper lamp intensity and clouds with high humidity and high concentrations; and dirty lamps may affect response.
Flame ionization detectors (FIDs) use a hydrogen flame to produce a specific type of carbon ion. Just as with the PID, ions allow flow of electrical current. They detect the low end concentrations of organic vapors and gases from 0.1 to 1,000 ppm. Limitations include low oxygen levels, cold temperatures and lower molecular weight hydrocarbon derivatives.
Gas chromatography
Gas chromatography (GC) sensor technology operates through differential separation (through the column) by the molecular weight of the contaminant. The mixture then passes into a flame ionization sensor. As different quantities of different molecularly weighted substances pass through the ionization chamber, their combustion is charted. Gas chromatography is used for the differentiation of mixtures, as well as the confirmation and identification of substances. It is also used for the identification of known possible contaminants. Limitations: operating temperature ranges average between approximately 45° F (7.2° C) and 105° F (41° C); unless one is using portable field units, one cannot provide readings in the field; gas chromatography is only intended to monitor organic compounds; and it requires a “footprint” (graph or GC run) on file to identify substances.
Ion mobility spectrometry (IMS) systems measure how fast a given ion moves in a uniform electric field through a given atmosphere. Sample molecules are ionized in numerous ways, including corona discharge (electrical arc) and radioactive sources. Samples of the ions are let into a drift chamber; once in the drift tube, ions are subjected to an electrical field. This electric field then drives the ions through the drift tube where they interact with the neutral drift molecules contained within the system. Chemical identification is based on the ion mobility (ion mass, size and shape) where they arrive at the detector for measurement. Ions are recorded at the detector in order from the fastest to the slowest, generating a response signal characteristic for the chemical composition of the measured sample. Ion mobility spectrometry is fast, highly sensitive, easy to use and compact. IMS can be used for the field detection of numerous chemical substances, including explosives, drugs and chemical weapons.
Surface acoustic wave (SAW) sensors are made by using a substrate material, quartz or lithium tantalate and bonded to a metal. This is then coated with a chemically absorptive material. Based on specific combinations of these materials and electrical charge, these sensors become highly sensitive to mechanical fluctuations (vibrations/acoustic waves) created by airborne substances attached to the surface. A surface acoustic wave makes for a very dependable and selective detector when multiple surface acoustic wave sensors with various absorptive coating are put in combination with pattern recognition software.
Spectroscopy in the field
Infrared spectroscopy detects the vibration characteristics of chemical functional groups in a sample. When an infrared light interacts with the sample, chemical bonds will stretch, contract and bend. Chemical functional groups tend to adsorb infrared radiation in a specific wave number range regardless of the structure of the rest of the molecule. The wave number positions where functional groups adsorb are consistent and not affected by temperature, pressure, sampling or change in the other parts of the molecular structure. Limitations: knowing what the substance is likely to be; must have footprint for known substances as a cross-reference; requires substantial maintenance and calibration; and interference gases can produce inaccurate readings. Infrared spectroscopy detects covalently bonded materials. It can be used to monitor one or more known substances and in the identification of compounds by comparison to known spectral data.
Raman spectroscopy technology is an excellent complement to infrared spectroscopy. Both Raman and infrared work well for identification of unknown substances. A higher percentage of positive identifications of unknown substances can be attained when both technologies are used. This leads to higher user confidence. Light (monochromatic/laser/single frequency) impinges upon a molecule and interacts with the electron cloud of the bonds of that molecule. The molecule will be excited from the ground state to a virtual energy state, and relax into a vibrational excited state, which generates scattering. Single frequency light is scattered at different frequencies and collected with an array detector. In order for the Raman to be sensitive, the sample must have a polarizable covalent chemical bond.
Radioactive materials
When radioactive materials are released, the human senses cannot detect radioactivity. The only way responders will know whether radioactive materials are present is with the use of instruments specially designed to detect radioactivity.
Four general types of radiation monitors are available for emergency response:
• Geiger counter – You can use a portable Geiger counter to detect radiation coming from samples in many different settings, from laboratories to mine shafts. It contains a cigar-sized, high-voltage vacuum tube, called a Geiger-Muller tube, that registers many kinds of ionizing radiation, including X-rays, gamma rays and alpha particles. The Geiger counter indicates radiation by making a clicking sound and showing the intensity level on a meter or digital display.
• Lithium fluoride crystal – People who work around radiation wear badges called dosimeters. The dosimeter keeps track of radiation they encounter on the job. A lithium fluoride crystal in the badge stores energy whenever ionizing radiation hits it. Technicians test the exposed crystal to make certain a worker’s radiation dose stays within safe limits.
• Bubble detector – Most radiation monitors cannot detect neutrons, so another device is needed for this kind of radiation. Bubble detectors serve as inexpensive, simple-to-use dosimeters. Neutrons produce tiny vapor trails in a special gel. The vapor forms bubbles that accumulate. The number of bubbles relates to the number of neutrons, which helps determine exposure to neutron radiation.
• Helium 3 detector – A helium 3 detector can monitor levels of neutron radiation much as a Geiger counter does for ionizing radiation. The high cost of helium 3 detectors limits their use to specialized laboratory and industrial applications.
Proper use
While knowing the technology behind monitoring instruments may be interesting and useful to some, it is “nice-to-know” information and not necessary to use the instruments appropriately. What is necessary is that responders know how to maintain, calibrate and properly use the monitoring instruments they have access to. They must also have the ability to select the proper instruments for the chemical(s) present when on the scene of a hazardous materials incident. The instrument manufacturer is the best source of information about specific monitoring instruments and important information for use and care.
ROBERT BURKE, Hazardous Materials and Fire Protection Consultant, a Firehouse® contributing editor, is a Certified Fire Protection Specialist (CFSP), Fire Inspector II, Fire Inspector III, Fire Investigator and Hazardous Materials Specialist, and has served on state and county hazmat teams. Burke is the author of the textbooks Hazardous Materials Chemistry for Emergency Responders, Counter-Terrorism for Emergency Responders, Fire Protection: Systems and Response and Hazmat Teams Across America. He can be contacted at [email protected].
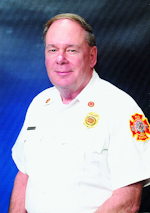
Robert Burke
Robert Burke, who is a hazardous materials and fire protection consultant and who served as a Firehouse contributing editor, is a Certified Fire Protection Specialist (CFSP), Fire Inspector II, Fire Inspector III, Fire Investigator and Hazardous Materials Specialist. He has served on state and county hazmat teams. Burke is the author of the textbooks "Hazardous Materials Chemistry for Emergency Responders," "Counter-Terrorism for Emergency Responders," "Fire Protection: Systems and Response," "Hazmat Teams Across America" and "Hazmatology: The Science of Hazardous Materials."